Interpreting Shifts in Blood Lactate Profiles
Understanding shifts in blood lactate profiles can provide insight into an athlete's physiological adaptations to training and help to interpret changes in performance.
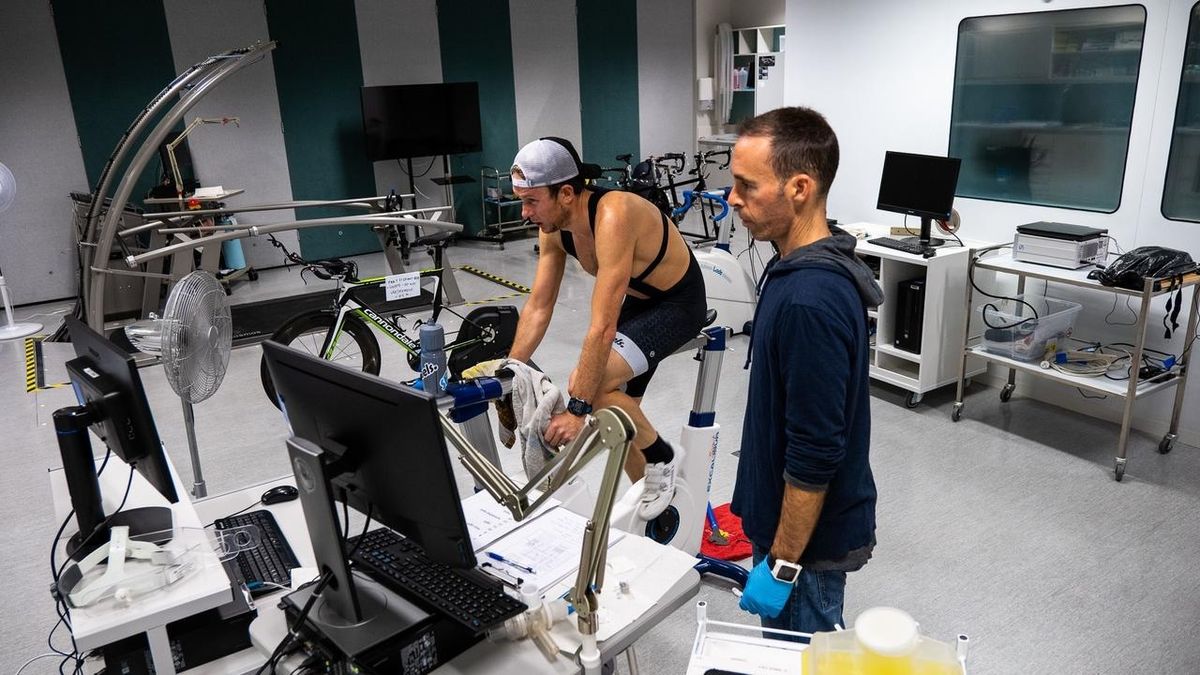
we are going to provide a little detail for athletes and practitioners on how to interpret changes in blood lactate parameters and profiles derived from serial laboratory-based lactate threshold assessments. These assessments typically involve exercising initially at a very low workload, considerably below the aerobic threshold, with heart rate and blood lactate concentration recorded prior to an increase in workload every 3-4 minutes (although these ‘steps’ can be longer). These steps are repeated until exhaustion. Expired gases may be collected throughout, and these can provide insight into movement economy, substrate utilization, and maximum oxygen uptake (VO2max). The tests are typically performed for (i) training zone identification and (ii) monitoring adaptations to training.
Classic rightward and leftward shifts
Below we have a textbook rightward-shift in the blood lactate vs. power output curve, which we would interpret as indicative of positive adaptation to a period of endurance training. This is because the power output at which the first rise in blood lactate concentration (or the “aerobic threshold”) has increased from 210 to 240 W, and the power output at which the exponential rise in blood lactate concentration occurs (or the “anaerobic threshold”) has increased from 300 to 330 W. Crucially, this is in the context of similar peak heart rates and blood lactate concentrations, and increased VO2max (Figure 1). Therefore, the athlete’s ‘range’ from a heart rate and blood lactate standpoint has remained constant, with an increase in overall aerobic capacity. Therefore, we can interpret the lower absolute blood lactate concentrations at given sub-maximal workloads as indicative of lower physiological stress.
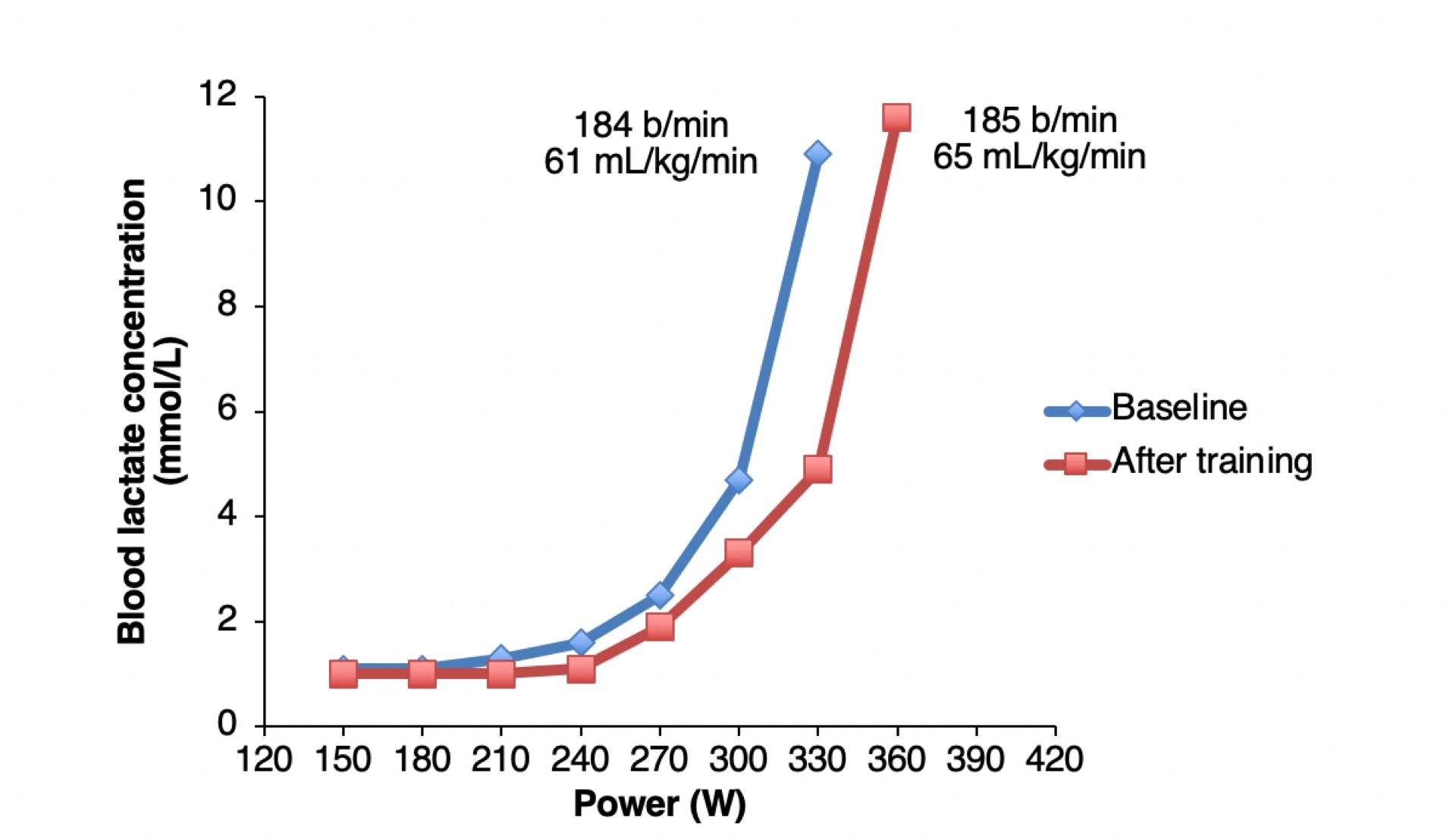
In contrast, below we have a textbook example of a leftward-shift in the blood lactate vs. power output curve similar to what we might observe after a period of detraining (Figure 2). The power output at which blood lactate concentration starts to rise has decreased from 210 to 180 W, and the power at which the exponential rise in blood lactate occurs has also decreased from 300 to 270 W. These changes in the blood lactate curve are accompanied by a decrease in VO2max, and similar peak heart rate and blood lactate concentration. Therefore, the athlete’s ‘range’ from a heart rate and blood lactate perspective is similar, but overall aerobic capacity has shrunk, so we can interpret the increased absolute blood lactate concentrations at given sub-maximal workloads as indicative of increased physiological stress.
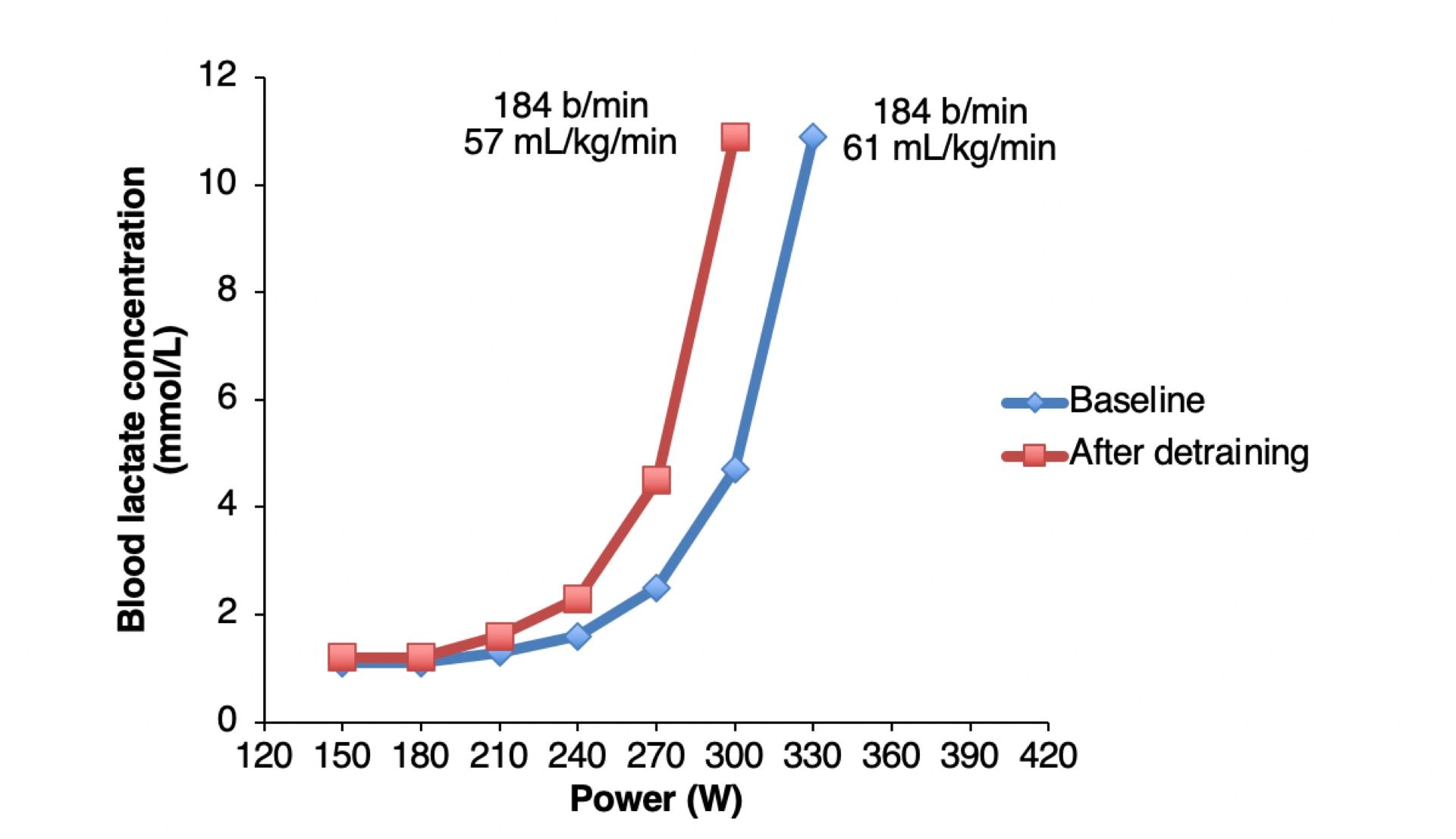
Overreaching
Now we are going to add a little nuance. A perhaps-overused term amongst endurance athletes is ‘overtrained’, and we add overused here because in our experience most athletes who appear ‘overtrained’ are actually probably acutely ‘overreached’, which is a subtle but important distinction. The overtraining syndrome is notoriously difficult to diagnose, and characterized by global fatigue and a reduction in exercise performance over a prolonged period (months). It is this time-frame, that the reduced exercise performance and wellbeing of the athlete is present for months, that is fundamental to the overtraining syndrome (5).
A much more common outcome of periods of solid endurance training is to become ‘overreached’. Becoming overreached may actually in many cases be an adaptive response to a period of high but tolerable training load. During a solid block of training, we are accruing positive adaptations to training that will help us out in the long-run, but also becoming acutely fatigued by all the recent training. When acutely overreached, it is possible that an athlete shows some sleep and mood disturbance and reduced motivation (2), and, critically in the present context, a reduction in maximum heart rate and decreased sub-maximal and maximal blood lactate concentrations (3, 4). Therefore, in an acutely overreached athlete, a reduced physiological ‘range’ can be evident (i.e. the highest observed blood lactate concentration or heart rate), and so a reduction in blood lactate concentration at a given sub-maximal workload may in fact not be indicative of lowered physiological stress as it is typically interpreted. One way of conceptualizing this is to imagine that if peak blood lactate concentration has decreased from 12 to 8 mmol.L-1, exercising with a blood lactate concentration of 4 mmol.L-1 is now 50% of maximum, rather than 33% as it previously had been. An example of a curve of an acutely overreached athlete is shown below (Figure 3).
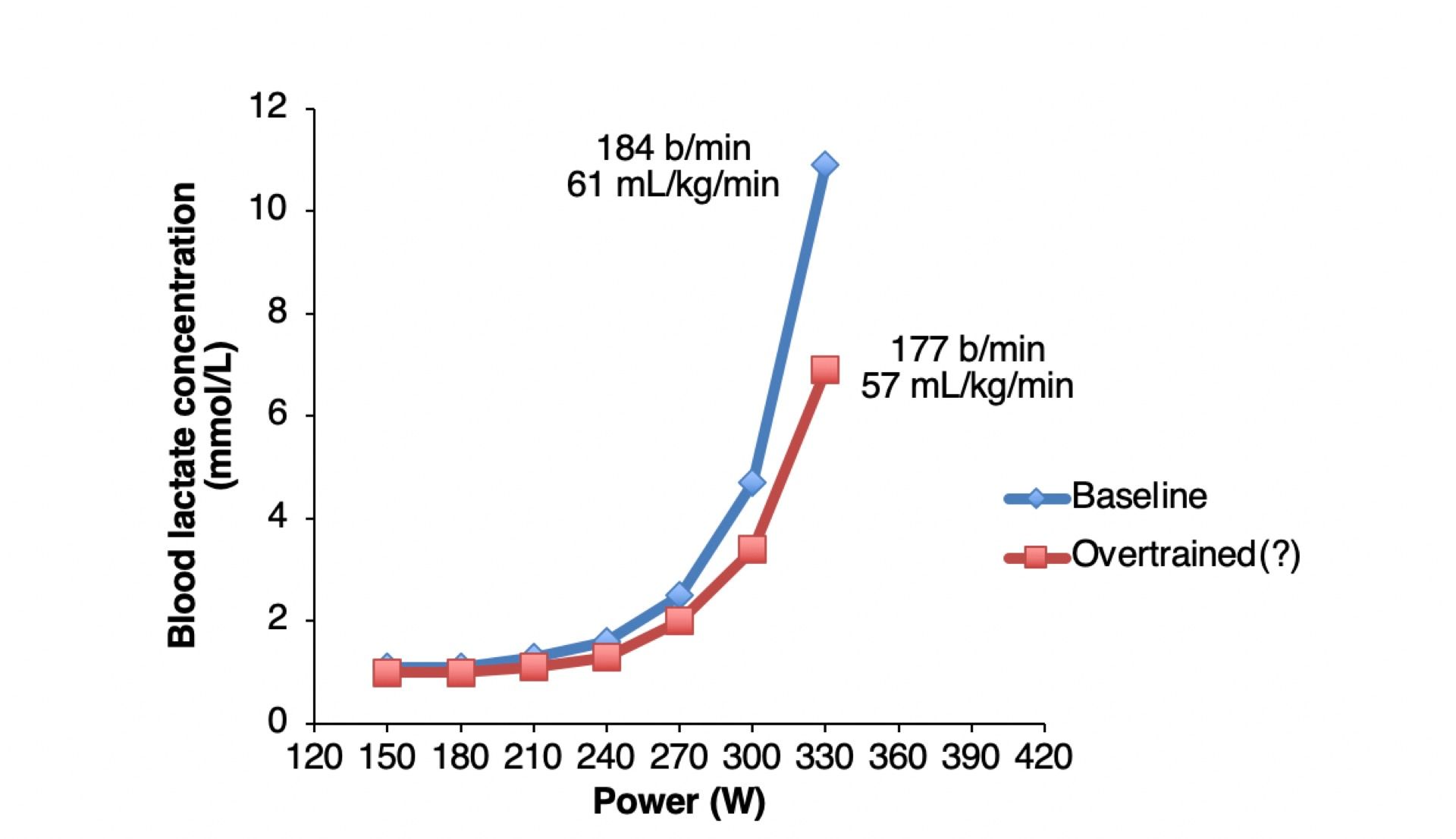
Therefore, if we test the athlete following a period of heavy training load before they have fully recovered from a training block, we may well see a reduction in peak heart rate and peak and sub-maximal blood lactate concentrations as described above. However, if we were to then test them again a week later, following a de-load to dissipate fatigue, we may well finally see the benefits of all those training adaptations, and observe a supercompensatory, positive rightward-shift in the lactate curve (Figure 4). This is the response we are hoping for with tapering, and you should take note not only of the reduced sub-maximal lactate concentrations compared to baseline, but the increase in VO2max and maintained physiological ‘range’.
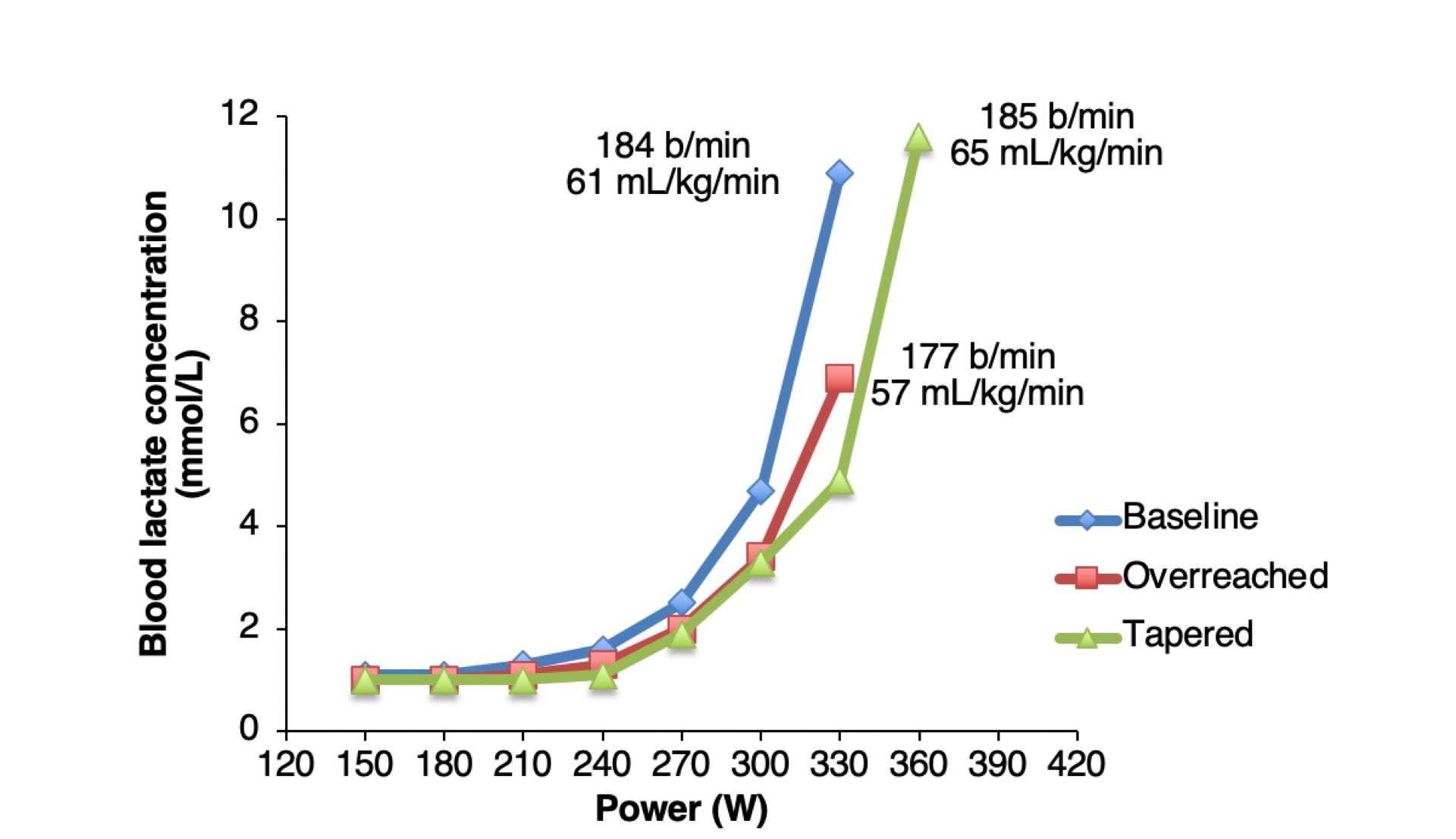
We are cautious to acknowledge that as athletes and practitioners, we are treading a fine-line here; if an athlete pushes too hard, or rather overreaches too far, they will typically fail to effectively absorb the actual benefits of their training, and feel increasingly miserable. We call this non-functional overreaching (5). However, if an athlete does not train enough, they are unlikely to maximize the adaptive benefits of their training. Therefore, finding the correct training dose that maximizes the adaptive benefits of training whilst maintaining athlete wellbeing is one of the main challenges faced by an endurance coach. This will of course vary between-athletes, and there is some data to suggest one key physiological trait that dictates the optimal training dose is muscle fibre type profile (1).
The period of time that an athlete is ‘overreached’, and how long the athlete is in a state of maladaptation, is critical. Training hard for any period of time when each training session is not effectively absorbed and positively adapted to is not optimal. Every athlete adapts and recovers very individually, which is why monitoring athletes and knowing the signs of overreaching (e.g. via heart rate variability (6), subjective measures, sleep quality) and applying recovery periods accordingly is the best approach to ensuring overreaching remains functional in the medium-to-long term.
Summary
Therefore, when interpreting data from blood lactate vs. power profiling tests, it is important to take note of the whole picture, rather than just the shape of the curve. Of course, you will want to look for the classically-defined thresholds, but assess and interpret these alongside the athlete’s VO2max, peak heart rate, and peak blood lactate concentration. These should all be considered in the context of how fresh and rested the athlete was when they performed the test, and the quality of their recent training sessions, perceived exertion, and wellbeing metrics.
References
- Bellinger P, Desbrow B, Derave W, Lievens E, Irwin C, Sabapathy S, Kennedy B, Craven J, Pennell E, Rice H, Minahan C. Muscle fiber typology is associated with the incidence of overreaching in response to overload training. J Appl Physiol 129: 823–836, 2020.
- Jeukendrup AE, Van Diemen A. Heart rate monitoring during training and competition in cyclists. J Sports Sci 16: S91–S99, 1998.
- Jeukendrup AE, Hesselink MKC. Overtraining - what do lactate curves tell us ? Br J Sports Med 28: 239–240, 1994.
- Jeukendrup AE, Hesselink MKC, Snyder AC, Kuipers H, Keizer HA. Physiological changes in male competitive cyclists after two weeks of intensified training. Int J Sports Med 13: 534–541, 1992.
- Meeusen R, Duclos M, Foster C, Fry A, Gleeson M, Nieman D, Raglin J, Rietjens G, Steinacker J, Urhausen A. Prevention, diagnosis, and treatment of the overtraining syndrome: Joint consensus statement of the european college of sport science and the American College of Sports Medicine. Med Sci Sports Exerc 45: 186–205, 2013.
- Plews DJ, Laursen PB, Stanley J, Kilding AE, Buchheit M. Training adaptation and heart rate variability in elite endurance athletes: Opening the door to effective monitoring. Sport Med 43: 773–781, 2013.